Computer simulations of accelerator materials for CLIC studies
The Compact LInear Collider(CLIC) is an electron-positron collider currently under development at CERN, Switzerland. The CLIC study aims at a center-of-mass energy range for electron-positron collisions of 0.5 to 5 TeV, optimised for a nominal center-of-mass energy of 3 TeV. It is planned to be a powerful successor of the LHC and well equipped for studies of e.g. the newly discovered Higgs boson.

Fields of CLIC studies applications:
- physics beyond the Standard Model;
- precise measurements of the Higgs boson;
- ancer cure;
- free electron lasers;
- vacuum circuit breakers;
- fusion reactors.
The construction of CLIC still requires certain issues related to material science and plasma physics to be properly understood. In order to achieve such high energies mentioned above and remain the accelerator compact (~50 km long), extremely high electric fields (100 MV/m and above) must be used. However, this conditions provoke vacuum arcs in the accelerating structures, also called breakdowns, that potentially lead to particle bunch loss. For the efficient operation of accelerating structures, the frequency of these breakdowns must be held under a very small value, less that 10-7 breakdowns/pulse. Thus, a proper understanding of the vacuum arc phenomenon would enable an accelerator design that mitigates this effect.
The vacuum arc formation phenomenon in the CLIC structure has been under study with a large range of different modelling techniques by the Doc. Flyura Djurabekova’s team at the University of Helsinki for many years. Our research group investigates the physical phenomena in the accelerator materials before, during and after the vacuum breakdown. The main research tools are computer simulation techniques like molecular dynamics, kinetic Monte Carlo and finite element method.
Particle-in-cell (PIC) simulations of plasma
The numerical modelling of plasmas requires methods that are quite different from e.g. methods that are used to simulate solids. The particles of interest in plasma systems are usually electrons, neutrals, and ions, and although these particles are not bound together like the particles in a lattice, they are intrinsically tied together through electromagnetic interactions. Thus, when describing plasmas, both microscopic and macroscopic information are equally important. For the modelling of plasma initiation in vacuum arcs, the particle-in-cell method has been applied. PIC is a commonly used method in the area of plasma physics that is based on a kinetic description using the Lagrangian approach. The kinetic approach is essential for the modelling of the transient plasma initiation phase, where the velocity distributions are far from Maxwellian. Moreover, only the self-consistent treatment of particle-induced and external fields of the PIC method can allow for an appropriate treatment of a system where high electric fields and boundaries (absorbing walls) are present.
Molecular Dynamics (MD) Simulations, HELMOD
The main hypothesis concerning the appearance of vacuum arcs is that short-lived field-enhancing asperities (or tips) are created due to surface or subsurface processes, such as diffusion or dislocation mechanisms, respectively, which result in a build-up of a plasma and a subsequent arc, i.e. burning of the plasma. An important question is how the tips are created. Molecular Dynamics (MD) simulations performed by the group using classical MD code PARCAS have shown that a void near the surface could nucleate dislocations that could be able to protrude the surface and subsequently create a tip. Growth of surface protrusion under the influence of a high electric field is shown below(for details, see A. S. Pohjonen et al. 2013, link below)
Links
We have developed a hybrid molecular dynamics – electrodynamics code HELMOD, based on the classical MD code PARCAS. In addition to the standard inter-atomic interactions, HELMOD also accounts for the effects of an applied external electric field on a metal surface. The code calculates the local electric field above the surface, which is enhanced compared to the applied field, and a partial charge is induced on surface atoms by the field. The code also considers resistive heating due to the resulting field electron emission current, and electronic heat conduction, which is not usually included in standard molecular dynamics. Using the code it is possible to simulate the dynamic evolution of surface features, such as field emitters, under the influence of high electric fields.
Kinetic Monte Carlo (KMC), KIMOCS
The long-term processes such a surface diffusion contributing to the tip formation can not be studied with MD due to the time limitations of MD. Insted Kinetic Monte Carlo approach can be used for the systems of the relatively big volumes. With this model, it is possible to study the long time scale evolution of the surface in absence of the electric field, such as what happens to the Cu sample in the time span after the electric field is turned off before the sample is studied in atomic force microscopy (AFM) or transmission electron microscopy (TEM) measurements.
Finite element method (FEM)
Finite difference method is utilized in electric field and temperature distribution calculations in and around the surface imperfections. Finite element method is used as preliminary development tool for atomistic simulations for quickly estimating the stresses both in the material and on its surfaces. Its future use will be to complement and to replace finite difference calculations. Molecular dynamics helps to investigate the material behaviour at atomistic level at fine details on small volume, kinetic Monte Carlo, on the other hand, catches coarser details on the relatively big volume.
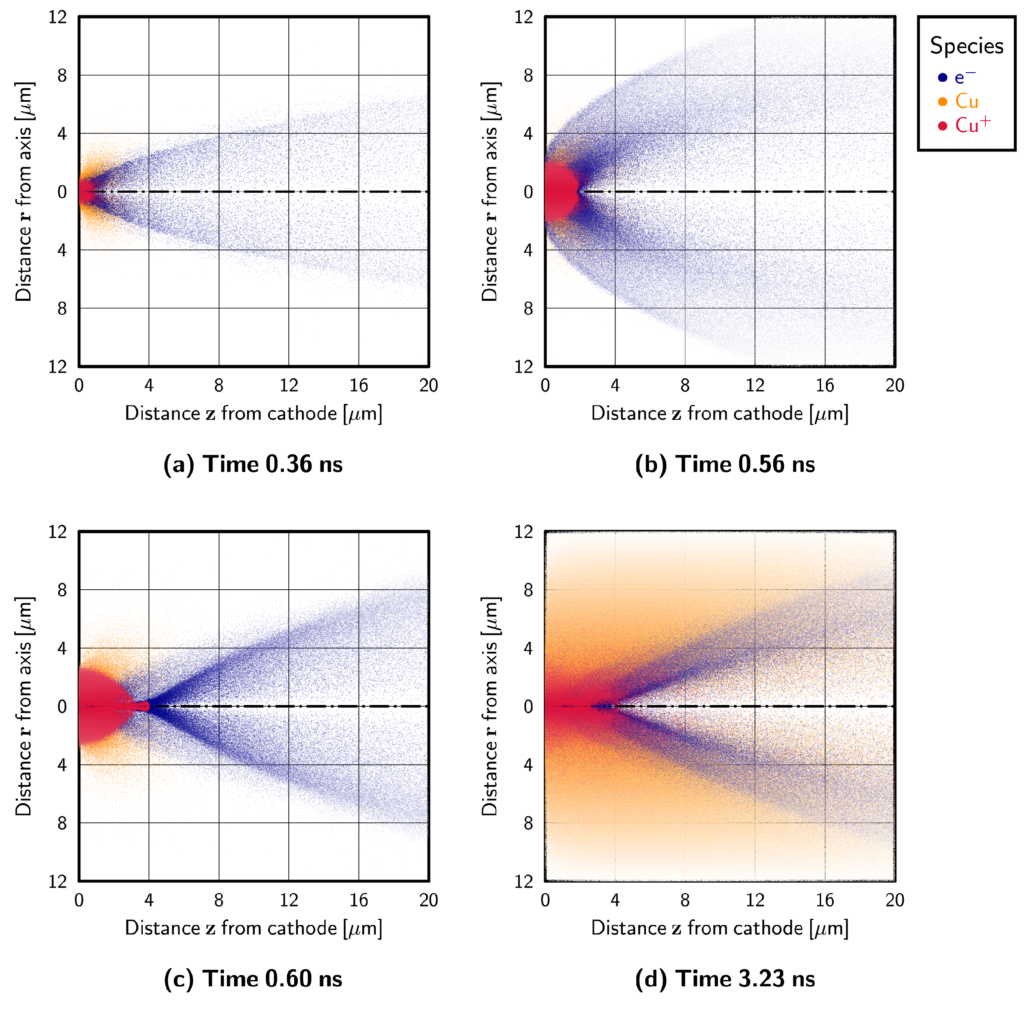